Heat Treatment
Heat treatment is a process of heating and cooling a material, typically a metal, to achieve specific properties such as improved strength, hardness, toughness, or ductility. There are several different methods of heat treatment, each of which is designed to achieve a particular set of properties.
The most common methods of heat treatment include annealing, normalizing, quenching, and tempering. Annealing involves heating the material to a high temperature and then allowing it to cool slowly, which helps to remove internal stresses and improve its ductility. Normalizing is similar to annealing, but the material is cooled in air instead of slowly, which produces a harder, stronger material.
Quenching involves rapidly cooling the material by immersing it in a liquid such as water or oil, which produces a very hard, brittle material that is highly resistant to wear and tear. However, this material is also more prone to cracking and failure, which is why it is often tempered.
Tempering is a process of reheating the material after it has been quenched and then allowing it to cool slowly, which helps to reduce the brittleness and improve the toughness and ductility. This produces a material that is strong, but also flexible and resilient.
Heat treatment is a critical process for many industries, including manufacturing, aerospace, and automotive, as it allows for the production of materials with specific properties and performance characteristics.
Objective of Heat Treatment
To increase strength, hardness and wear resistance (bulk hardening, surface hardening)
To increase ductility and softness (Tempering, Recrystallization Annealing)
To increase toughness (Tempering, Recrystallization annealing)
To obtain fine grain size (Recrystallization annealing, Full annealing, Normalizing)
To remove internal stresses induced by differential deformation by cold working, nonuniform cooling from high temperature during casting and welding (Stress relief annealing)
To improve machinability (Full annealing and Normalizing)
To improve cutting properties of tool steels (Hardening and Tempering)
To improve surface properties (surface hardening, high temperature resistance- resistanceprecipitation hardening, surface treatment)
To improve electrical properties (Recrystallization, Tempering, Age hardening)
To improve magnetic properties (Hardening, Phase transformation)
Classification of steels
Compositions, such as carbon (or non alloy ), low -alloy, and alloy steels
Manufacturing methods , such as converter, electric furnace, or electroslag remelting methods
Application or main characteristic, such as structural , tool, stainless steel, or heatresistant steels.
Finishing methods, such as hot rolling, cold rolling, casting, or controlled rolling and controlled cooling.
Product shape, such as bar, plate, strip, tubing, or structural shape Classification of steels
Oxidation practice employed, such as rimmed, killed, semikilled, and capped steels.
Microstructure, such as ferritic, pearlitic, martensitic, and austenitic
Required strength level, as specified in the American Society for Testing and Materials (ASTM) standards.
Heat treatment, such as annealing, quenching and tempering, air cooling (normalization), and thermo-mechanical processing.
Quality descriptors and classifications, such as forging quality and commercial quality.
Allotropic Transformation in Iron
Iron is an allotropic metal, which means that it can exist in more than one type of lattice
structure depending upon temperature. A cooling curve for pure iron is shown below:
Effect of Pressure on Allotropy of Iron
Iron - Cementite phase diagram
The Fe-C (or more precisely the Fe-Fe3C) diagram is an important one. Cementite is a metastable phase and ‘strictly speaking’ should not be included in a phase diagram. But the decomposition rate of cementite is small and hence can be thought of as ‘stable enough’ to be included in a phase diagram. Hence, we typically consider the Fe-Fe3C part of the Fe-C phase diagram.
In the phase diagram, temperature is plotted against composition. Any point on the diagram therefore represents a definite composition and temperature. The phase diagram indicates the phases present and the phase changes that occur during heating and cooling. The relative amounts of the phases that exist at any temperature can also be estimated with the help of lever rule.
A portion of the Fe-C diagram – the part from pure Fe to 6.67 wt.% carbon (corresponding to cementite, Fe3C) – is technologically very relevant.
Cementite is not a equilibrium phase and would tend to decompose into Fe and graphite. This reaction is sluggish and for practical purpose (at the microstructural level) cementite can be considered to be part of the phase diagram. Cementite forms as it nucleates readily as compared to graphite.
Compositions upto 2.1%C are called steels and beyond 2.1% are called cast irons. In reality the calssification should be based on ‘castability’and not just on carbon content.
Heat treatments can be done to alter the properties of the steel by modifying the microstructure→ we will learn about this in coming chapters.
Fe-Fe3C metastable phase diagram
Carbon Solubility in Iron
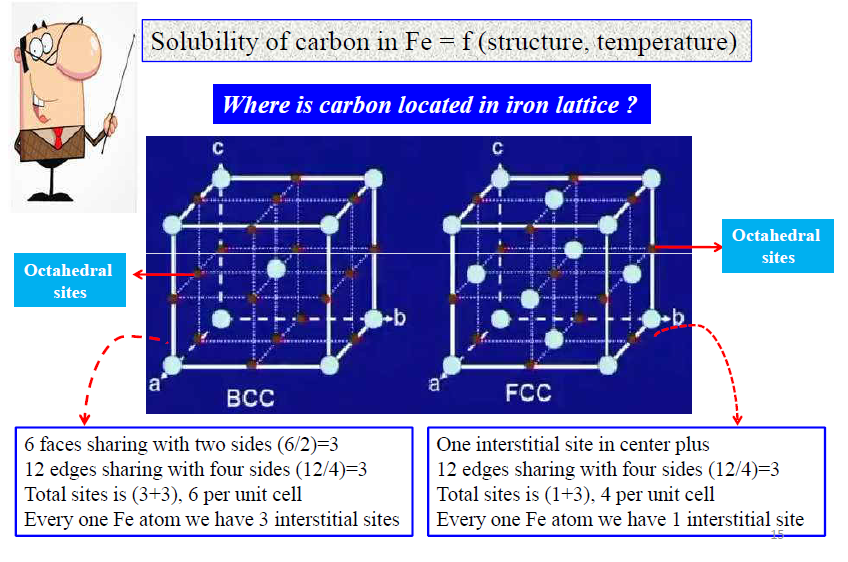
Why concentration of carbon in α-Fe with BCC structure is less than γ-Fe with FCC structure?
FIRST LET US CONSIDER FCC STRUCTURE (γ-Fe)
Packing factor of FCC lattice 0.74
This indicates the presence of voids. Let us discuss it more elaborately.
In a FCC crystal there are mainly two types of voids:
Tetrahedral: coordination number (CN) is 4. That means the void is surrounded by 4 atoms.
Octahedral: CN is 6. That means the void is surrounded by 6 atoms.
There are 8 tetrahedral voids in a unit cell. That means 2 voids per atom.
There are 4 octahedral voids in a unit cell. That means 1 void per atom. Why concentration of carbon in α-Fe with BCC structure is less than γ-Fe with FCC structure?
However, impurities prefer to occupy octahedral voids.
Because the ratio of the radius of the tetrahedral void to atom is 0.225 and the same for the octahedral void is 0.414.
The ratio of the radius of the carbon atom (size is 77 pm) to Fe (when it has FCC crystal) is 0.596.
So when a carbon atom occupies any void, lattice will be distorted to increase the enthalpy.
Distortion will be less if it occupies the octahedral voids.
Although it increases the enthalpy, carbon atoms will be present up to a certain extent because of the gain in entropy, as explained previously, which decreases the free energy.
Carbon Solubility in Iron

Why concentration of carbon in α-Fe with BCC structure is less than γ-Fe with FCC structure?
NOW LET US CONSIDER THE BCC UNIT CELL (α - Fe)
Packing factor of BCC lattice 0.68. So total void in a BCC unit cell is higher than FCC cell.
However, there are 12 (6 per atom) tetrahedral and 6 (3 per atom) octahedral voids present. This number is higher than the number of voids present in a FCC unit cell.
Unlike voids in FCC lattice, in a BCC lattice the voids are distorted. That means if an atom sits in a void, it will not touch all the host atoms.
The ratio of the radius of tetrahedral void to atom is 0.29 and the radius of octahedral void to atom is 0.155.
The ratio of the radius of the C atom (size is 77 pm) to Fe (when it has BCC crystal) is 0.612. Why concentration of carbon in α-Fe with BCC structure is less than γ-Fe with FCC structure?
So it is expected that in a BCC unit cell, impurities should prefer tetrahedral voids.
However, although the octahedral void size is small, planar radius which has 4 atoms on the same plane is 79.6 pm, which is larger that the C atom size. That means it needs to distort only other two atoms.
On the other hand if C sits in the tetrahedral void it has to distort all four atoms. So in α – Fe with BCC unit cell C occupies the octahedral voids
Now the octahedral void size in g-Fe (FCC) is higher than a-Fe (BCC). So naturally the distortion in a BCC cell will be higher and the activation energy for impurities to occupy a void in a BCC cell also will be higher.
This is the reason that we find much lower solubility limit of C in a-Fe.
Why carbon preferentially sits in the apparently smaller octahedral void in BCC ?